Gene therapies have a big problem. Here's what you need to know
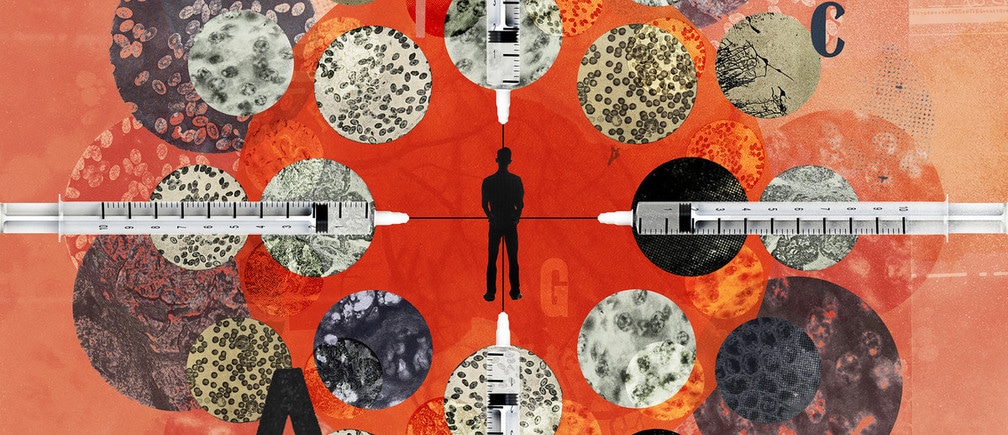
A solution for some, but not for all. Image: Eleanor Shakespeare for Mosaic
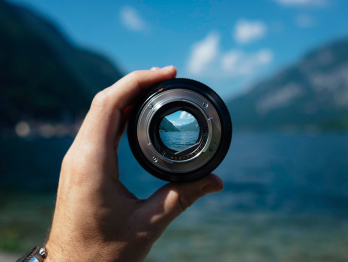
Get involved with our crowdsourced digital platform to deliver impact at scale
Stay up to date:
Healthcare Delivery
Growing up in Devon in the 1980s, brothers James* and Matt* had to be careful with their antics – a cut or bruise could land them in hospital.
They have haemophilia, a genetic disease where the blood doesn’t clot efficiently due to a lack of a clotting protein. It’s plagued their family for generations. Their granny’s brother died from it, and they, their uncle and a younger cousin have long managed it by injecting the missing protein to prevent bleeds.
While haemophilia can now be managed effectively – in high-income countries at least, where medication is readily available – it can severely limit freedom and quality of life and can even reduce life expectancy. Bleeds can be triggered by minor strains that healthy people barely notice, such as from walking or lifting boxes. Blood then seeps from the capillaries and pools in the joints, causing throbbing pain and lasting damage.
Although the younger of the two at 41, Matt’s joints are the more wrecked; he can’t straighten his right arm due to eroded cartilage in the elbow, while both his ankles and his right knee are in bad shape. For James, 45, it’s worst in his ankles, which swell up and bleed after walking or standing for too long.
So when they heard about a clinical trial for an experimental gene therapy, both brothers were intrigued. Unlike the injections, the therapy might fix their haemophilia for good. It’s a simple idea: if you inherit a faulty gene, then putting a working copy into the body’s cells should fix things.
We might at long last be on the cusp of a new era in which it will be possible to halt certain genetic disorders. In 2012, the EU approved its first therapy targeted at a specific mutated gene, one which causes liver disease; five years later, a genetic treatment for vision loss was approved in the USA. There are ongoing clinical trials of gene therapies for dozens of other diseases.
“Gene therapy has the potential to be transformational,” says David Schaffer, whose lab at the University of California, Berkeley, researches gene therapeutics. The best available drugs for diseases like haemophilia have only transient effects, he says, but using DNA as a medicine means that “the field, at least ambitiously, is thinking of a cure”.
For people like Matt and James, these new treatments could be life-changing.
There’s just one problem. For many of us, they won’t work.
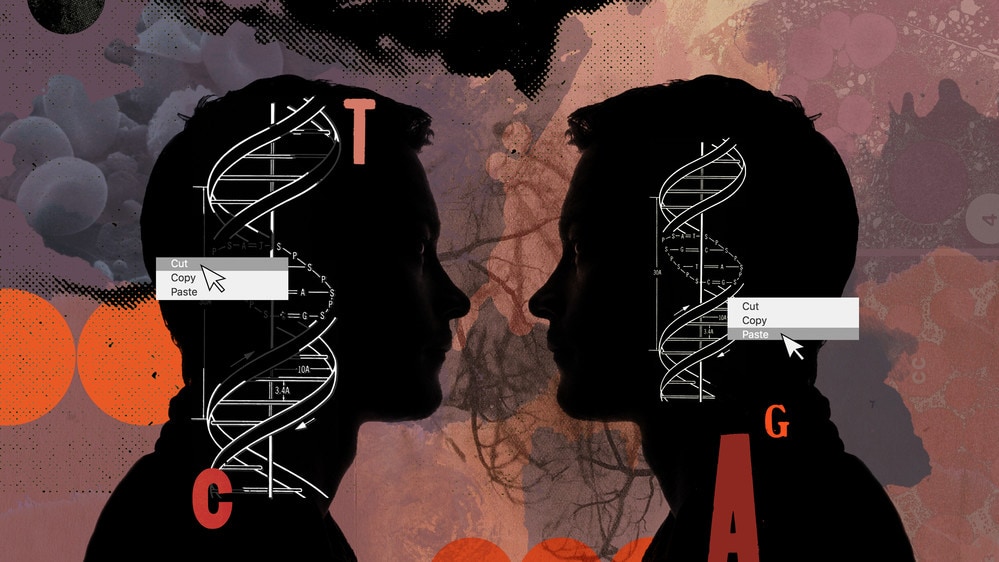
Even for siblings, James’s and Matt’s worlds are alike. They both live in East Anglia, not far from each other, and have friends in common and go to the same pubs. Both are fans of indie music, and have had to endure crippling pain from standing at live gigs. Outside of work, James is the frontman of a local post-punk band, and Matt used to play guitar in another group.
And they both have haemophilia A, caused by a genetic mutation that makes their bodies unable to produce a blood-clotting protein called factor VIII. It affects about 1 in 5,000 men worldwide – it’s much, much rarer in women because the faulty gene is located on the X chromosome and is recessive, meaning its effects are usually masked if the body has another working copy. Women typically have to have a mutated gene on both their X chromosomes to suffer the effects.
“As kids, we played outside a lot,” says James. “The house where we grew up had a massive playing field behind it and an abandoned quarry. I remember us going to hospital a lot because of a bleed or a sore joint, bad cuts, a bash on the head.”
While James accepted his condition and did his best to not get hurt, Matt was more reckless, splitting his head open more than once as he rebelled against having to be careful. “When I was younger, I tried to ignore the disease,” he says. “That’s probably why I have more joint issues.”
When they got hurt, their parents would rush them to the doctor to receive injections of factor VIII, purified from donor blood. It wasn’t until their 20s that prophylactic injections of factor VIII became available to them. Life got easier: spontaneous bleeds could be avoided; joint damage could be controlled.
But they still had to boost their factor VIII every three to four days, meaning the injections would always fall on different days of the week, something that had to be kept track of. And they’d take 30 minutes each – far from ideal.
The gene therapy would instead give their bodies a working gene for them to make their own factor VIII. But transferring a gene into a person is no easy feat. Genes are long strings of DNA that don’t readily get inside cells. So therapies typically rely on viruses, which squirt DNA into cells’ nuclei as a way of life. In this case, a virus, modified so that it doesn’t cause disease, and with the healthy human factor VIII gene added to its DNA, is injected into the body. It then enters the body’s cells to deposit its cargo, leaving it as a separate piece of DNA in the nucleus next to the host cell’s genome. The cells then start using the implanted gene to create factor VIII.
After all those years of being forced to be careful, Matt decided to sign up for the clinical trial. But James was unsure – he didn’t fancy having to undergo lots of follow-up testing. He decided to speak to one of his uncles, who also has haemophilia. “He comes from an era when there was no treatment,” says James. “He said I should just give it a go.”
Part of a larger ongoing trial, it was a small study, with only 15 participants, and by the time James had also decided to enrol, all the spots had filled up. But soon afterwards, he got a call letting him know that a place had become available. A person had dropped out. It was Matt.
A blood test had revealed Matt had antibodies for the adeno-associated virus, or AAV, that the trial was using as the therapy’s carrier. A chance event – a fleeting infection earlier in life – had rendered him immune to the treatment. As Matt withdrew from the study, James took his place.
Matt is not alone. Across the population, it’s thought that immunity to AAV5, the specific type of virus used in this study, could be as high as 50 per cent; for other AAV types it could be higher still – for AAV1 and AAV2, perhaps as much as 70 per cent. But estimates vary, and without a standard way to test for immunity, we don’t really know the scale of the issue. What we do know, says Schaffer, is that pre-existing immunity is the main reason volunteers are excluded from gene therapy trials, and that it will severely restrict who gets to be treated if and when these therapies become widely available.
Luk Vandenberghe, a gene therapy researcher and associate professor of ophthalmology at Harvard Medical School, says that immunity to AAV is gene therapy’s Achilles heel. These are “incredibly potent therapies,” he says, “but they are only going to be relevant to a sliver of the population”.
So why, then, transport genes with such a common family of viruses that so many people have been exposed to?
The truth is that AAV wasn’t originally the leading candidate for gene delivery. It only came to the forefront after a tragic event that would change the field for ever.
The idea that genes could be used as medicine began brewing in the second half of the 20th century, drawing on new insights into the structure of DNA and the way that genes code for proteins.
It was clear early on that gene delivery would be difficult, but the knowledge that viruses embed their genetic material in cells raised the possibility that they could be co-opted as delivery vehicles. The development, in the 1970s, of tools for cutting and stitching together DNA finally made this possible.
The first trials to transfer genes into humans began in the late 1980s and 1990s. Yet the preferred vectors weren’t AAVs but retroviruses and adenoviruses; the latter, despite their similar-sounding name, are very different from AAVs. Adenovirus’s big draw was that it was easy to engineer and grow in the large quantities needed for treatment.
A concern was that a patient’s immune system might react badly. Adenovirus was known to elicit an immune response, but researchers considered this a manageable risk. They were wrong.
On 13 September 1999, Jesse Gelsinger, an 18-year-old from Arizona, received an infusion of adenovirus in a gene therapy trial to treat a urea cycle disorder. He soon developed a fever and other reactions to the virus. Some other trial participants had also reacted to the virus, but none as severely as Jesse. His immune system unleashed an all-out attack that could not be stopped. He slipped into a coma and died of multiple organ failure four days after receiving the treatment.
The trial, led by James Wilson of the University of Pennsylvania, was promptly stopped. A subsequent investigation by the US Food and Drug Administration (FDA) found mistakes in its execution, including patients not meeting eligibility criteria and the researchers failing to inform patients of adverse reactions previously seen in animals. In a wrongful death lawsuit, the Gelsinger family also alleged that Wilson had inadequately disclosed a conflict of interest, as he had a stake in a biotech company that would have profited from the trial’s success. Wilson was banned for five years from sponsoring FDA-regulated clinical trials or participating in research on human subjects, and the legal cases brought by the Gelsinger family and the government against the investigators and university were settled out of court.
Gelsinger’s death cast a long shadow over the fledgling field, and threw considerable doubt over whether adenovirus could be used for these sorts of therapies.
With heightened concern about patient safety, it was AAV’s time to shine.
First discovered in the 1960s as a contaminant of adenovirus, AAV will always remain linked in name to its notorious predecessor. But unlike adenovirus, which is a cause of the common cold, AAV sweeps through the body silently without signs of infection.
Jude Samulski, today the director of the Gene Therapy Center at the University of North Carolina, isolated the first AAV genome in the early 1980s while a graduate student at the University of Florida. This allowed researchers to start thinking about engineering it into a gene delivery tool.
But it was difficult to grow, and adenovirus remained the popular choice. AAV, says Samulski, “was considered a stepchild”.
By the mid-1990s, though, it had been shown that AAV could safely shuttle genes into lab animals, and that the genes then worked. AAV had become a serious candidate for gene delivery. It was just unfortunate that, at the time of the Gelsinger trial, research with adenovirus was at a more advanced stage.
But after the tragedy, it was clear that a much less obtrusive virus was needed. Gene therapies require pumping massive doses of the carrier virus into people – many times more than you’d see in a natural infection. At such high doses, a virus that doesn’t normally cause an immune response is far less likely to get out of hand and harm the patient.
“With adenovirus, you are taking a pathogenic virus and you’re attempting to turn it into a harmless FedEx truck for the genes,” says Samulski. “AAV was a natural FedEx truck.”
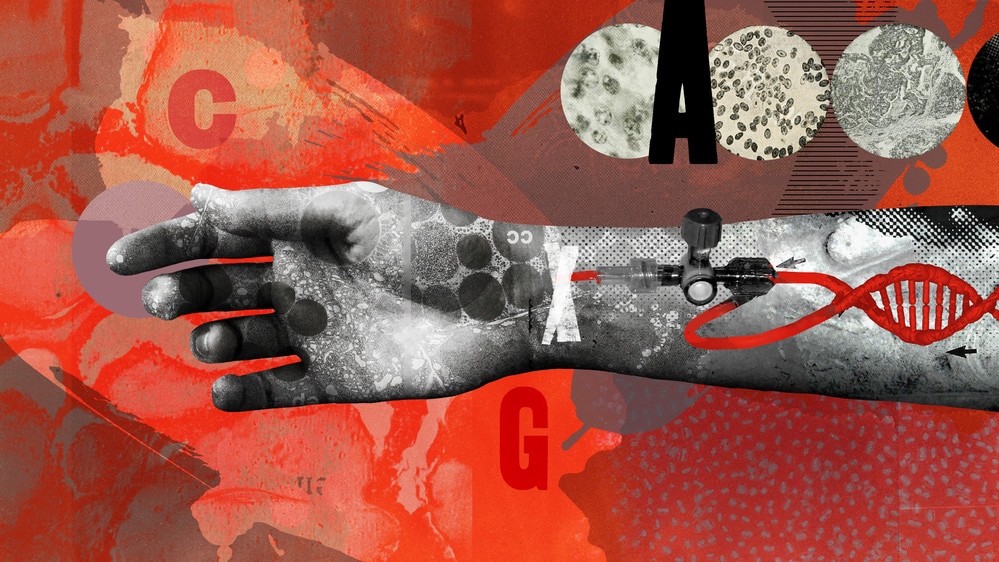
James’s therapy was scheduled for a Monday in April 2016, at a hospital in west London.
The timing wasn’t ideal. He had recently bought a house with his partner, Anna*, and the move ended up falling just three days after his treatment. Frayed and exhausted from packing, the couple took the Sunday afternoon train to London, where they spent the night.
The next morning, James gave himself one last injection. The doctors told him to hold off putting in any outside factor VIII after this, to make it easier to detect if and when his body started making its own. If the treatment failed, his joints would start hurting within a couple of days. But even as his body was about to be genetically altered in a procedure that not long ago belonged to science fiction, James had other worries.
“No one was sure when the gene therapy would start working,” he says. “They just told me to be a bit careful. I was like, ‘Well that’s well and good, but I’ve got to move house later this week.’”
The treatment itself was a mere hour-long IV drip. This is how long it took to inject James’s arm with a cup full of liquid containing over 450 trillion AAV virus particles, each carrying a working copy of the factor VIII gene. Once in the bloodstream, some of the virus would find its way into James’s liver and the cells lining his circulatory system, where factor VIII is normally made, and unload its cargo.
During the next 24 hours, James was not allowed to leave the treatment room, and he was closely watched for any adverse effects. There were none. The room had a chair for Anna to sit on, a TV and a bathroom off to the side, but for James, being confined in a tight space was the worst part of the treatment.
The next day, the couple went home and moved house as planned.
“I could already feel something,” says James. “I remember sitting down at the end of the day after putting all that furniture together, and I did not feel quite so bad. And then the next day we were properly moving and I carried loads of boxes. I was tired but otherwise feeling good.”
Before, this level of exertion would make his joints flare up with fresh bleeds. But five days after the treatment, blood tests revealed what he had suspected – his body was making its own factor VIII. The therapy was working.
The trial had started with two patients receiving a low dose of the treatment. When this proved safe, six others were given an intermediate dose, and with this proving safe too, a further seven – including James – got a high dose. Nine of these patients were included in the trial’s write-up. It’s what’s known as a dose-escalation study.
The low-dose patients saw no improvements, and the intermediates some, but it was in the high-dose patients where the effects were most pronounced – all bar one went on to produce their own factor VIII at levels seen in healthy people. Their rates of spontaneous bleeds dropped, as did their need for injections.
“When we started seeing these levels, which were essentially normal… it just blew it out of the water. We were completely gobsmacked,” says John Pasi, professor of haemostasis and thrombosis at Barts and the London School of Medicine and Dentistry and the lead investigator of the study, which was funded by BioMarin Pharmaceutical.
“Because it was so painless, it seemed so easy,” adds James. “Do 24 hours in London, and that was it. It changed my life – it’s crazy.”
When he was rejected from the trial, Matt hadn’t been that upset. But seeing the effects on his brother, the significance of it began to hit home. “If I knew how good the outcome would be, I would have been disappointed,” he says. “Obviously I’m disappointed now.”
James took part in an early phase of the trial, to test the therapy’s safety and efficacy. The study has since expanded to test efficacy on a larger number of patients. Following the US FDA’s announcement last year that it would streamline its review process for emerging gene therapies, Samulski thinks the first one for haemophilia could become widely available within three years.
Two and a half years after the treatment, James’s factor VIII levels still hover in the normal range. He has not used a syringe since he gave himself that last injection just before the gene therapy. By all accounts, he appears cured.
The Gene Therapy Center occupies two floors of a modern red-brick building on the western rim of the University of North Carolina campus at Chapel Hill, which is lush with greenery on the May morning that I visit. Exams have just ended and the campus, normally full of students, is empty. Jude Samulski, the director, is walking with a slight limp due to a back injury from a few months before. “I fought the Christmas tree, and I lost,” he says.
During his time there, the Gene Therapy Center has created myriad AAV vectors, which are being tested for a host of diseases, including ones that affect the muscles, brain and blood.
Samulski himself has continued to perfect gene delivery both in his lab and through start-ups. One of these – Bamboo Therapeutics – was recently bought by Pfizer, along with an AAV vector that it equipped with a version of a muscle gene called DMD. Pfizer is now testing this vector in people with Duchenne muscular dystrophy, a devastating condition where the muscles degenerate, caused by a lack of dystrophin, the protein DMD codes for.
Another large pharmaceutical company, Novartis, announced in April 2018 that it had acquired AveXis, a start-up behind a promising gene therapy for spinal muscular atrophy, a similarly devastating disease that, in its most severe form, is often fatal in early childhood.
That pharmaceutical giants are getting into the field signals that gene therapy, long plagued by the 1999 tragedy, is finally being taken seriously, Samulski says.
But this isn’t helping people like Matt. “The clinical research hasn’t tipped the scales for patients who have been excluded: if you are fortunate, you can get treated; if you are not fortunate, you can’t,” Samulski explains. “The field is now moving towards finding ways to overcome the remaining challenges, including pre-existing immunity.”
And the clues to this lie in the virus itself.
A viral particle is composed of a protein shell, or capsid, with its genetic material tucked inside. What exactly these viral proteins do is a mystery, and one of the many virologists trying to unlock it is Lauriel Freya Earley, a postdoctoral researcher at Samulski’s lab.
Earley has an encyclopedic knowledge of AAV. The capsid, she tells me, consists of three proteins – vp1, vp2 and vp3 – which somehow self-organise into an icosahedral, or 20-faced, structure that is 3,000 times smaller than the width of a human hair.
She hands me a piece of plastic that looks like a starfish, its surface rugged with tiny spikes. It’s a piece of a 3D-printed model of a blown-up AAV capsid. Besides shielding the viral DNA, the capsid also acts like a key to help the virus get inside different organs in the body. Bits of protein material stick out to form a bumpy surface, which – somehow – helps the virus enter cells.
In the 37 years since Samulski cloned the first AAV, which came to be known as AAV2, a number of other types have emerged. There’s estimated to be around a dozen that can infect human cells, and a number of these are being tested as gene therapy vectors for different diseases. The types are distinguished by their different capsids, which allow them to do different things. AAV5 and AAV8, for example, breeze into the liver. AAV9 can access the liver as well, and it can also cross the blood–brain barrier, an obstacle for many molecules, and so could deliver genes to the brain. As well as sending genes to different tissues, the diverse AAVs could also provide a way around the immune system.
The capsids also make viruses draw the fire of the immune system. When someone who has been exposed to a virus develops antibodies to it, it’s these distinctive structures that they target for destruction.
While the majority of people may have already been infected with AAV2 – studies with higher estimates suggest around 70 per cent of people have antibodies – it’s thought that exposure is lower for other AAV types. For people like Matt, who are immune to one, there’s hope they could be treated with another.
Researchers are also creating new variants in the lab. One way to do this is to engineer capsids so that they are no longer recognised by antibodies. This would be straightforward if researchers knew which bits of the capsid antibodies recognise, but that’s another part of AAV biology shrouded in mystery.
An alternative approach is to create many, many mutant capsid variants, and then select those that slide past the immune system unnoticed.
“There’s no reason that we should be at the mercy of whatever viruses nature has thrown over the fence at us,” says David Schaffer of the University of California, Berkeley, who is also a founder of 4D Molecular Therapeutics, a start-up for AAV design. He believes we should engineer AAVs, both to optimise them as gene delivery vehicles and to bypass the immune response. “If we make enough changes in the surface coat of proteins, we can evolve the virus so it’s no longer recognised by the pre-existing antibodies.”
A few years ago, Samulski applied this approach to a real-life situation. Six boys with Duchenne muscular dystrophy had taken part in an early-stage trial using a modified form of AAV2 as a vector for gene therapy. The aim had been not to treat them but to test whether this AAV2 was safe and effective at delivering the relevant gene – and it was. But, as a result, the boys had all developed high levels of AAV2 antibodies, which would preclude them from receiving another dose as treatment in the future.
So Samulski’s team collected blood from three of them, exposed a vast collection of mutated AAV2 capsids to the antibodies it contained, and selected any that evaded detection. AAV2 coated with these capsids could, in principle, be used to deliver therapies to these three boys. However, Samulski’s experiment was only an academic exercise to show that the approach worked.
Under current regulations, personalised gene therapy remains unrealistic. Regulatory agencies do not distinguish between naturally arising and artificially created AAV capsids, so each new variant has to go through onerous and costly clinical trials, says Samulski. “But given that thousands of patients are being excluded overall, there has to be some common-sense conversation between regulatory agencies and clinical need.”
However, even if the approval process were to become more streamlined for artificial AAVs, not everyone is on board. James Wilson, who has shifted the focus of his research from adenoviruses to AAVs, is concerned that engineering AAVs too much could turn a harmless virus into something more pernicious. Instead, he’s focused on isolating new natural AAVs from primates.
“These exotic AAVs are sufficiently different not to be recognised by the immune system,” Wilson says. “But are they good vectors? That’s what we’re working on now.”
Samulski, though, is undeterred by criticism of artificially creating viruses.
“You can look for natural viruses, but if you know how to evolve them, then that’s the same. Natural does not mean better. There is no evidence that engineered viruses could be dangerous.”
On a Friday night in April 2018, a few days short of the second anniversary of his treatment, James’s band performed at a pub in Cambridge. Before, he would have had to boost his factor VIII levels to be on top form, but this was no longer necessary.
The constant worries about having to be careful with his body and to remember the injections have disappeared. He and Anna can now plan longer holidays together without having to think about how much medication to bring along, or whether it will be possible to store it in a cool place.
“I feel like a different person,” he says. “I don’t even think about it. It’s weird how very quickly you get used to not doing something you’d done for 43 years.”
The treatment hasn’t caused friction between the brothers, and they remain close. James, though, admits that he feels guilty about the trial’s success, and having something Matt doesn’t. There’s a sadness too for both over a bond no longer there. Throughout the course of their lives, they had tested different medications together and always had each other to talk to about their disease. They still talk about it, but it’s not the same as before.
No one knows for how long James’s new factor VIII gene will work, but an earlier gene therapy trial for haemophilia B offers hope: patients are continuing to produce their own clotting factor eight years later.
But James does worry about his levels dropping. “In the back of my mind, I’ve got the feeling that this might not last for ever, so I’m totally enjoying it while it lasts. I even have totally irrational thoughts, like maybe I should worry about my levels more, instead of taking them for granted – that way they might stay higher.”
If James were to lose his modified cells as they regenerate, he probably wouldn’t be able to repeat the treatment, because it’s likely he too will now be immune to it. And because gene therapy requires giving high doses of the carrier virus, his AAV5 antibodies are far more plentiful than those produced in a natural infection. This might preclude him from treatment with other AAV types.
But in the future, we may not even need viruses for gene therapy. Researchers are looking to develop other delivery methods, from liposomes – fluid-containing fatty molecules that can fuse with the cell’s membrane – to synthetic nanoparticles that won’t be seen by the immune system. Researchers are also investigating gene-editing methods to permanently embed the working gene into the recipient’s genome.
“There’s lots of funding in gene therapy research, so problems will be easier to solve. It’s only a matter of time,” says Samulski.
As it turns out, Matt doesn’t have to wait long. Since he first applied to take part in the trial, BioMarin has changed how it determines immunity. A subsequent test found Matt’s AAV5 antibody levels to be below threshold, making him eligible for the study’s later stages.
It’s a surprising turnaround – and a reminder that, despite its history, the field is still in its infancy, grappling to fully understand the challenge of pre-existing immunity. “If all goes well, I should be starting the trial in about six months, so obviously I’m very pleased,” Matt says.
It also shows, says Samulski, the need to standardise immunity tests – as everyone at the moment is experimenting with different methods. Until we understand exactly what level of immunity hinders treatment, we might be unnecessarily denying people life-changing therapies.
“Gene therapy is in such early days,” he says, “we are still testing what is going to work.”
* Some names have been changed.
Mosaic contacted BioMarin for comment, but it said that as it operates under the Association of the British Pharmaceutical Industry code of practice it would be unable to provide specific information about the haemophilia A treatment discussed in this piece until it is licensed and approved.
Don't miss any update on this topic
Create a free account and access your personalized content collection with our latest publications and analyses.
License and Republishing
World Economic Forum articles may be republished in accordance with the Creative Commons Attribution-NonCommercial-NoDerivatives 4.0 International Public License, and in accordance with our Terms of Use.
The views expressed in this article are those of the author alone and not the World Economic Forum.
The Agenda Weekly
A weekly update of the most important issues driving the global agenda
You can unsubscribe at any time using the link in our emails. For more details, review our privacy policy.
More on Health and Healthcare SystemsSee all
Katherine Klemperer and Anthony McDonnell
April 25, 2024
Vincenzo Ventricelli
April 25, 2024
Shyam Bishen
April 24, 2024
Shyam Bishen and Annika Green
April 22, 2024
Johnny Wood
April 17, 2024