The big yet small future of science
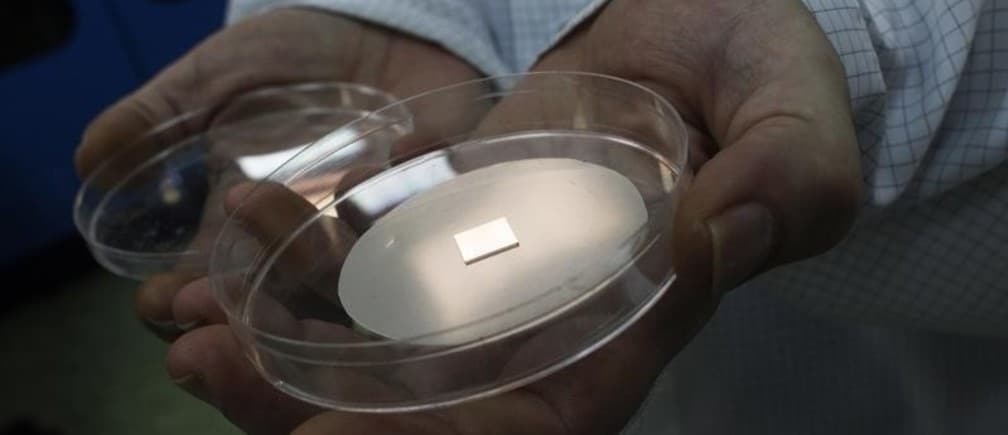
Image: Senior technologist Dariusz Czolak holds a piece of silicon carbide disk covered with a layer of graphene, obtained in the process of epitaxy, in the Institute of Electronic Materials Technology (ITME) laboratory. REUTERS/Kacper Pempel.
French-born astrophysicist Dr France Córdova has long shattered glass and cultural ceilings. She and her 11 siblings grew up with little exposure to the sciences. But just after earning a bachelor’s degree in English from Stanford, Dr Córdova saw a US public television special on neutron stars. Compelled to learn more, she made her way to the office of a Massachusetts Institute of Technology researcher featured on the programme… and left with a job.
Since then, Dr Córdova has become a rocket scientist, a wife and mother, the youngest and first woman to become chief scientist at NASA, the first female president of Purdue University and, since 2014, director of the National Science Foundation. The $7bn agency funds scientific research across the United States. In this Future scope, Dr Córdova shares her insights into what’s on the horizon in science and tech research. Some of the answers have been edited for brevity and clarity.
What do you see as the most pressing innovation challenges for 2016?
There are many pressing innovation challenges, but if I were to pick one, I would say the need to scale up the US innovation ecosystem and make it more evenly distributed geographically. Innovation shouldn’t just be centred in certain areas of the country. It should be everywhere, so that everyone has a chance to participate in the innovation economy and reap its benefits.
Big data and analysis-based modelling are becoming more and more critical to basic research. Could you tell us a bit more about how this has affected your work?
Big data is, indeed, becoming important in fundamental research. The possibilities of scientific study are now expanding and researchers are gaining access to vast new data sets and analysis tools. Due to that shifting landscape, NSF does more than just support projects that integrate big data into existing fields—we fund research that looks at big data’s potential and ways to avoid misusing it.
For example, we fund a number of high‑performance computers, such as a new one called Wrangler. (It’s in Texas, of course.)
Wrangler is designed for data‑intensive science. It assists researchers with problems ranging from market analysis of stock options to combating human trafficking to astronomy surveys.
Then there’s Arizona State University’s NSF-funded Decision Center for a Desert City, which has a huge bank of 360-degree roundup computers. It reminds me of mission control. Big data analysis combined with behavioural analytics helps researchers analyse climatic uncertainties, urban-system dynamics and adaptation decisions.
For instance, they can bring up different areas of the state and view where the water is coming from. How is it changing this year from 10 years ago? What are their energy needs? What kind of crops are they growing? Where are those going? Are they feeding locally or are they feeding outside the state? They can also identify which crops are producing the highest yield for biofuels. Can we restore marginal or polluted lands for growing plants for biofuels? How can we harness the power of enzymes to break down stalks and other inedible materials for biofuels? What are the best new methods for refining biofuels?
This kind of basic, bottom-of-the-food-chain, fundamental research, which is the kind we fund, is essential to eventually creating products.
Some 50bn things are expected to be connected by 2020. How do we ensure that the resulting massive increases in data are not misinterpreted or misused, especially when it comes to individuals’ information like health or consumption patterns?
This is a very important topic and universities are especially forward-thinking here. They want their students to understand that there is an ethics component to the technologies.
Responsibility in science has always been a part of having a humanistic view of what you’re doing. Synthetic biology, for example, opens up such questions.
I’m an astrophysicist by training. That field has been using big data since the first research satellite was launched. There’s always the concern that data be properly interpreted.
I used to worry about my graduate students in that regard. They’d put something in one end of a computer program and out would pop a result. I’d say, “Well, let’s just do a back‑of‑the‑envelope calculation and see if it makes sense.” An area of research is developing to ensure that big data doesn’t create fake discoveries.
We’re also looking at ways to keep big data secure. Some of the scientists we fund are developing data analytics, management methods and technologies that are meant to anonymize data when it involves personal information, keep it tracked and protected. It offers such a world of opportunities, but we know we have to be careful. It’s a scary world, and it’s not just about people looking at our data and worrying about how to protect it. To that end, we’re also funding research that focuses on how hackers and cyber criminals communicate. The idea is that if we understand their social systems, we can better predict likely targets.
Let’s explore energy a bit. What do you see as important developments on that front in 2016 and which ones are you focusing on?
At the University of Texas at Austin, a research team led by the inventor of the lithium-ion battery, Professor John Goodenough, is developing a safe, sustainable cathode material for low-cost sodium-ion batteries. Sodium, in contrast to lithium, is abundant and inexpensive and could one day be used for wind and solar energy storage. The team’s new cathode material is made of the nontoxic and inexpensive mineral eldfellite, a promising development towards creating a commercially viable sodium-ion battery.
We’re also funding research into studying the vulnerability of smart grids. Local variabilities like your Internet-connected television set or refrigerator can open the door to hacking. Again, the research is interdisciplinary. Aside from computer scientists, we have social scientists looking at the behavioural side of the smart grid and the changing relationship between the power grid and customers.
Let’s turn to advanced manufacturing. What’s new on the horizon?
We’re investing in research that aims to create cyber‑enabled adaptive manufacturing systems. Machine learning—machines learning as they go—can improve processes. And we’re using a lot of nano‑scale manufacturing research.
For example, a project we’re funding at the University of Illinois at Urbana-Champaign involves transfer printing, a type of nanomanufacturing. Nanocircuits are “printed” like tiny stamps. Think of the material used to make the circuit as the ink, which might be silicon or another semiconductor. The stamp transforms the ink into a nanostructure; together, a group of those transferred to a surface with other electrical properties then creates a circuit.
But when something’s that tiny, it’s hard to get the ink to come off the stamp. The researchers use lasers to break the adhesive forces, which could lead to faster printing of these tiny circuits. Those circuits could be used, for example, in a wearable so that an asthmatic person could monitor surrounding air quality.
We’re investing in other areas with real potential, from 3D printing to smarter manufacturing systems that use machine learning to adapt and conserve resources. We’re also pushing the frontiers of new materials. For example: The world of high-powered electronics requires components made with very high thermal conductivity. Our supported researchers are learning how to layer materials like graphene to make them suitable for those applications.
Here again, we see challenges; some nanomaterials and some 3D-printed materials can be toxic. We’ve funded research that tests for those kinds of dangers.
Nano also holds promise for personalised medicine, both in the diagnostics and in the treatment of diseases such as cancer. What kinds of advancements do you think we could see in the next few years?
I have in front of me a little peptide array—in plexiglass, so I can’t use it—developed at ASU’s Biodesign Institute. You put different blood drops in and they can read immunosignatures to tell if you have one of five different types of cancer.
I asked the lead investigator, “Did you apply to NIH for funding?” They said it was too high‑risk. Instead, we funded it. A lot of what we fund is considered “high‑risk, high-reward”: People don’t know what the outcome will be or where the research will go. But fundamental science enables the kinds breakthroughs that drive the economy. We’ve had 217 supported researchers go on to win Nobel prizes—including two of this year’s laureates in chemistry and economics—and some of the discoveries we’ve funded have generated billions of dollars in economic impacts.
Personalised medicine is a hot topic. Here, again, we see big data and machine learning. We fund a project at John Hopkins University using both to treat complex, chronic autoimmune diseases. The system learns patterns that can indicate whether certain treatments improve or worsen symptoms, thus helping doctors design individualised treatment plans.
Then there’s nano: Vanderbilt University just announced that a NSF‑supported engineering team has developed hardware and software designs for medical-capsule robots. They made it open source, allowing researchers around the globe to create customised ones.
The idea is that within years they’ll be pill‑sized. You ingest them, and they can perform tasks like screenings, diagnosis and event treatments while they’re inside of you.
What would be your recommendation to young minds interested in science today? And why should they push through barriers and pursue it?
There’s no such thing as a career, or a calling, without obstacles. Some challenges can be invigorating—embrace those. Once you take a challenge apart, overcoming it a piece at a time, you see that there’s no goal you can’t achieve. Be persistent. Build a life shaped by you and no one else.
Of course, there are some challenges that can be more vexing than just those associated with doing the best you possibly can in your field. There are societal pressures and the prejudices of people and institutions. You have to surmount those, like any other obstacle.
We know these barriers exist and are studying why they persist and how we can bring them down. We’re also working to ensure that all students receive adequate scientific education, even if they’re not planning on careers in the sciences. In an increasingly technology-driven workforce, this kind of knowledge is more important than ever.
NSF is committed to the idea that everyone has a fair chance to pursue scientific learning. So, as you move forward, know that you’re not alone.
Don't miss any update on this topic
Create a free account and access your personalized content collection with our latest publications and analyses.
License and Republishing
World Economic Forum articles may be republished in accordance with the Creative Commons Attribution-NonCommercial-NoDerivatives 4.0 International Public License, and in accordance with our Terms of Use.
The views expressed in this article are those of the author alone and not the World Economic Forum.
Stay up to date:
Innovation
The Agenda Weekly
A weekly update of the most important issues driving the global agenda
You can unsubscribe at any time using the link in our emails. For more details, review our privacy policy.
More on Emerging TechnologiesSee all
Hope French and Michael Atkinson
November 7, 2024